
In the first four reports in this series, we examined Sustainable Agriculture (SA) and Sustainable Intensive Agriculture (SIA) in a global framework of both greenhouse gas (GHG) emissions and the need for increased food production. In the fifth report, we started to look at arable farming and now continue on the same theme with a discussion of nitrogen usage in an arable setting.
The Importance of Nitrogen
Nitrogen is a critical nutrient for plant growth through the process of photosynthesis, the process through which plants use carbon dioxide and water to create their own food.
Although air contains nitrogen, plants cannot absorb it and therefore have to acquire it from root uptake in the soil. This soil nitrogen rapidly becomes depleted and applied synthetic fertiliser compensates for this, although some plants can also produce their own nitrogen through the process of fixation.
Plants also need other nutrients divided into macro nutrients (phosphorus, potassium, and sulphur) as well as a range of other nutrients such as calcium and magnesium, and a range of micronutrients such as zinc and boron. Plants are extremely responsive to nitrogen but will also often require additional applications of other nutrients to keep the balance correct in the growing plant.
In the 1970s and 1980s, agronomists all over the world were chasing yield and production. Take the case of wheat. When a new recommended list of wheat varieties appeared such as by the UK National Institute of Agricultural Botany (NIAB) or the Canadian Alberta Regional Variety Advisory Committee (ARVAC), the first attributes agronomists often considered were resistance to lodging or ‘standing power’ or other similar terms, rather than overall yield. The reason was that most of the varieties of feed grade wheat (milling wheats are slightly different) were within a few percentage points of each other on yield and this could easily be compensated for by using more nitrogen fertiliser.
The wisdom of the time (Graph 1) was to use as much nitrogen as needed to get to the optimum nitrogen application rate. Because the fertiliser was inexpensive, this was very close to the maximum theoretical yield. Assistance also came from a range of growth regulating agrochemicals to increase the standing power as well as a good dose of other agrochemicals to compensate for disease which was often more prevalent in high-yielding crops. Above a certain point, the crop will lodge (fall over) causing a drop in quantity and quality especially because of harvesting difficulties. We also used the plough and other tillage equipment with wanton abandon.
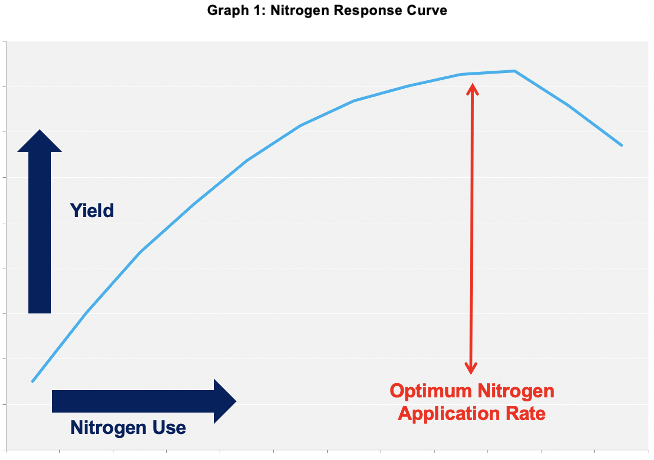
The result of this was that from 1960 to 2000, global synthetic nitrogen usage increased by over 750%.
The problem with this approach is that it leads to environmental consequences – GHG emissions, wastage, and adverse consequences for the water supply, as unused nitrogen finds its way into this by processes such as runoff and leaching.
However, producing dwarf hybrid crops with good standing power is still important because this characteristic can protect against lodging for another reason, namely from the weather, such as high winds and excessive rainfall.
The question then arises, can we somehow dial back the amount of nitrogen we use or the way we use it in the field without losing or even gaining yield? Is there a different farming system we can use to improve this undesirable system? The answer is yes.
GHG Modelling
There is a valuable tool, primarily intended for farmers, that has been developed by Cool Farm Alliance. It’s free and allows the user in any country to put in field-by-field details, such as soil characteristics, temperatures farming method, inputs, fuel use (on farm and to the point of delivery of the harvested crop), irrigation, etc.
It is available for all main crop and livestock uses, with separate sections for potatoes and rice. It then calculates the greenhouse gas emissions for the described situation and allows ‘what if’ scenarios by changing the variables.
What makes this tool and its algorithms so useful is it takes account not only of events on the farm but also the fertilizer factory production figures and all fuel use. It also breaks out the different types of greenhouse gases, rather than just quoting one figure as CO2 equivalent.
There are other similar models available and they don’t always produce the same results, but the direction is mostly similar. For example, I set it up for a 20-hectare (ha) UK feed winter wheat crop, producing 8 tonnes/ha and using 625 kg/ha of broadcast 33.5% granular ammonium nitrate on a well-drained, and relatively dry, silty soil of moderate soil organic matter with a pH of 5.5 to 7.3.
Field operations were conventional tillage including ploughing. All other inputs (non-nitrogenous fertilizer, spray regime, etc.) were typical of current practice and there was no wastewater. No irrigation was used, and the crop was harvested dry (15% moisture). Transportation to the first point of sale was 75km using a Heavy Goods Vehicle (HGV). All fuel use was based on diesel (DERV) or diesel equivalents.
These were the results that provide our base (calibrated) level:
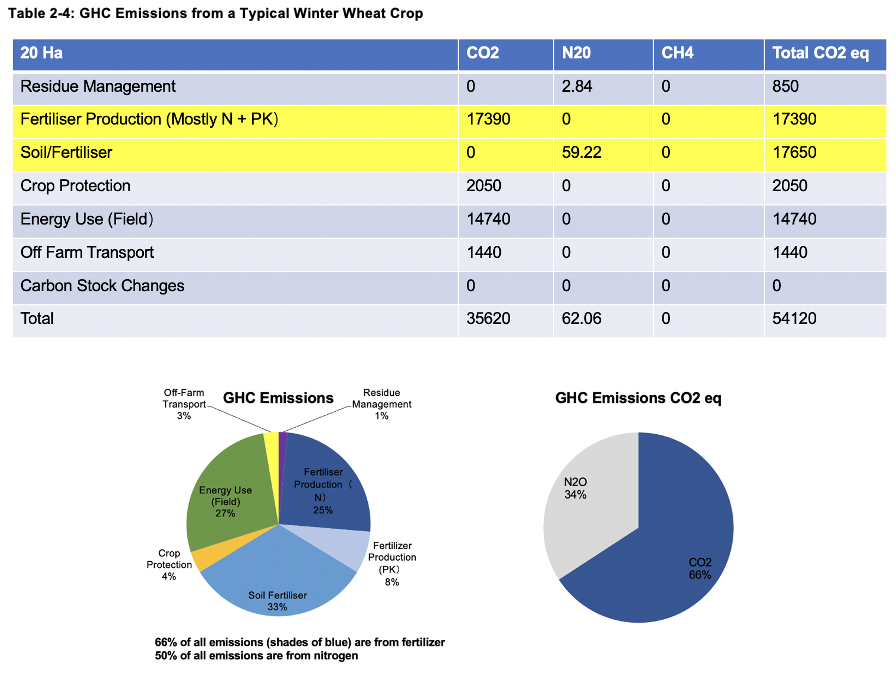
It’s important to remember, as we discussed my first report, that N20 is much more harmful as a greenhouse gas than CO2, which is why the conversion from N2O to CO2 eq (equivalent) is so dramatic. When broken down in a different way, after fertilisation, the energy, processing and fuel in-field component is also a significant contributor to GHG, in this case around 27% of the total.
Nevertheless, the fertilizer component, and in particular nitrogen, is clearly highlighted as the major problem (in yellow in the first table above).
It is now possible to examine changes to farming practice.
There are some things it is impossible to change, apart from selecting another field or a different crop. Changing the soil type to sandy or clay, or having a moist rather than dry soil, all increase GHC emissions by more than 10%. The science behind this is very complicated, although we will refer to it in future reports. Equally, GHG emissions reduce on extremely acidic soils, but the damage to yield is so great that remedial action (using lime or similar) is necessary.
We can now start to examine some changes in farming practice and their implications for GHG:
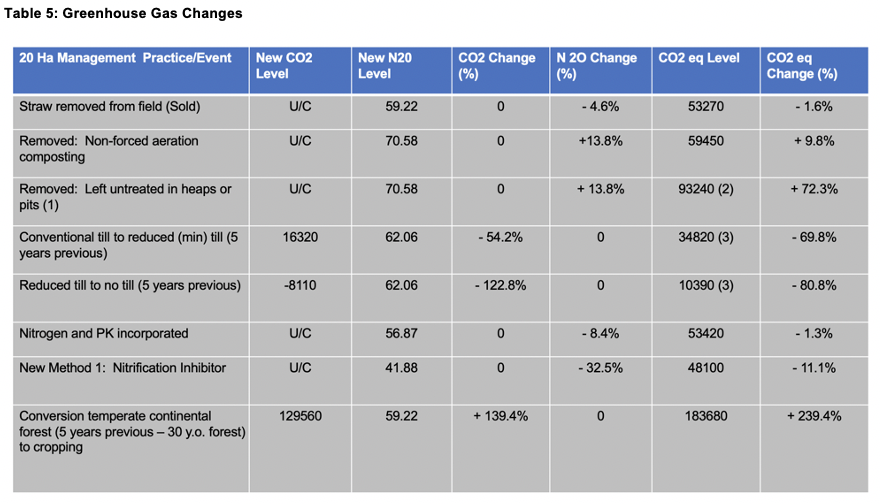
- This is not a common practice in the UK, but in some countries, it is more commonplace.
- Methane (CH4) = 33,790 CO2 eq
- From carbon sequestration
It can easily be observed why minimum or no till systems have become so important in this type of crop, with expensive ploughing a luxury only used in, for example, a four-year rotation of winter barley, oilseed rape, winter wheat, winter wheat, then plough and repeat. This is necessary to reduce the wheat volunteers in the next barley crop.
The examples in Table 5 are just a few of our future discussion points. Some are shocking, such as the removal of trees, and some offers a vision of the future.
New Method 1: Nitrification Inhibitor is just one of many new ideas (and some old ones) we will discuss in future reports, including nitrogen stabilizers, variable rate technology, fertilizer specific placement, balanced crop nutrition, rotation, injection systems, fertigation, and others. In some cases, we can combine practices/events for an enhanced effect.
Conclusion
Our actions on GHG emissions can have major positive and negative consequences.
So far, we have explored how to significantly reduce GHG emissions by some nitrogen manipulating methods.
In every case discussed, we have no loss of yield, which is consistent with SA, but it is not consistent with SIA, where we also need to increase yields.

Other Opinions You Might Be Interested In…
- NEW! Sustainable Agriculture: Origins and Evolutions
- Sustainable Intensive Agriculture: A New Approach
- Sustainable Agriculture: Arable Farming
- Which Fertiliser is Cheapest Today? 11th November 2020
